Table of Contents
- Why Stability Testing is CRUCIAL for Biologics and Cell Therapy Products
- Key Stability Challenges in Cell Therapy
- Regulatory Requirements for Stability
- Ultimate Guide to Post-Thaw Viability & Functional Integrity in Cell Therapies
- Container Selection and Its Impact on Cryopreservation and Stability
- Types of Stability Studies
- Conclusion: A New Paradigm for Cell Therapy Stability
- References
Why Stability Testing is CRUCIAL for Biologics and Cell Therapy Products
Stability testing reveals how critical quality attributes (CQAs)—from protein conformation to cell viability and function—respond to real‑world stresses. By mapping degradation pathways, manufacturers establish scientifically justified shelf lives, storage, shipping, and administration parameters that ensure product integrity from bench to bedside.
Biologics, including cell therapies, are inherently complex and structurally delicate. Their biological activity depends not only on the correct amino acid sequence (primary structure) but also on the preservation of higher-order structures—such as secondary, tertiary, and quaternary configurations—maintained through intricate non-covalent and covalent interactions (e.g., hydrogen bonds, hydrophobic interactions, disulfide bridges).
Even small changes in environmental conditions can destabilize these structures, leading to loss of function or increased immunogenicity. Common degradation mechanisms include:
- Deamidation of side chains (Asn, Gln),
- Oxidation of sensitive residues (Met, Cys, Tyr, etc.),
- Denaturation, where the protein loses its functional 3D structure,
- Aggregation, forming insoluble or immunogenic complexes,
- Glycosylation-related instabilities, such as the hydrolysis of sialic acid residues in glycoproteins.
Cell therapies add an additional layer of complexity, as the product is composed of living cells whose viability, phenotype, and function are easily disrupted by stressors such as temperature shifts, pH changes, or mechanical agitation. Unlike proteins, cells can undergo apoptosis, lose potency, or exhibit unintended behavior if their stability is compromised.
To maintain product performance, T cell therapy culture solutions, NK cell therapy culture solutions, and serum-free MSC culture media are increasingly used in cell therapy manufacturing. These provide consistent environments for cell growth and reduce variability tied to animal-derived components.
Due to these sensitivities, stability testing is essential to ensure that biologics and cell therapy products maintain their safety, purity, and efficacy throughout manufacturing, storage, and distribution. Regulatory authorities mandate rigorous stability programs to:
- Justify product shelf life,
- Define storage and shipping conditions,
- Support clinical trial material release (e.g., IND/IMPD),
- Safeguard patient outcomes in real-world settings.
Ultimately, without robust stability data, there is no assurance that the product administered to the patient is equivalent in quality and performance to the material tested in preclinical or clinical studies.
Did you know?
Shelf lives can be as short as 24 hrs!
Key Stability Challenges in Cell Therapy
While ICH Q5C covers recombinant protein-based biologics, several overarching principles—such as the need for real-time, real-condition data, attention to external stressors, and a lack of suitability for retesting—are directly applicable to cell therapy products. However, cell therapies introduce added layers of complexity that exceed the scope of traditional biologics.

1. Live Cells as Active Ingredients
ICH Q5C emphasizes the importance of characterizing purity and potency over time. In cell therapy, this becomes especially challenging because:
- Cells are not inert molecules—they are living systems whose viability, phenotype, and function (e.g., cytotoxic activity, cytokine production, or proliferation potential) change over time and under stress.
- Any environmental condition that affects these parameters may reduce therapeutic efficacy or increase the risk of off-target effects or immune reactions, which cannot be easily reversed or “retested” later.
Thus, per ICH Q5C, all factors impacting quality and potency must be considered—but for cell therapies, this requires multi-parametric assays (e.g., flow cytometry, metabolic function tests) far beyond what is required for proteins.
2. Short Shelf Life (Especially for Fresh Products)
ICH Q5C calls for long-term stability studies under intended storage conditions. For fresh, non-cryopreserved cell therapies, this is particularly difficult because:
- Shelf life may be only 24–72 hours, making real-time long-term studies impractical.
- Storage conditions are tightly constrained, often requiring controlled ambient temperatures (e.g., 2–8°C or even room temperature with strict time limits).
- In contrast to recombinant proteins, where shelf life can often be 1–5 years under frozen or refrigerated conditions, cell therapy shelf life is more fragile and time-critical, requiring real-time release testing and stringent cold chain logistics.
3. Cryopreservation and Freeze/Thaw Effects
While ICH Q5C supports the development of stability protocols based on real and accelerated conditions, the non-linear degradation kinetics seen in cell therapies post-thaw violate some assumptions behind classical models:
- Viability and potency loss may be disproportionate to time or temperature changes after thawing.
- Freeze/thaw studies must account for recovery kinetics, changes in membrane integrity, and phenotypic drift, which are not issues in protein therapeutics.
As a result, developers often rely on GMP-compliant cryopreservation media, DMSO-free cryopreservation media, and protein-free freezing media for primary cells to enhance cell recovery and mitigate variability.
Therefore, cell therapy stability testing must include post-thaw hold time validation, and ideally, assessment of whether viability and potency remain within specification throughout the entire in-use window.
4. Non-Standard Containers and Complex Matrices
ICH Q5C allows flexibility in study design but assumes relatively standard container-closure systems (vials, prefilled syringes). In contrast:
- Cell therapies are often stored in gas-permeable infusion bags, custom vials, or closed-system bioprocessing containers.
- Complex matrices may include infusion media, cryoprotectants (e.g., DMSO), cytokine supplements, or feeder cells, all of which may interact with the product over time.
To address this, cell factories for bioproduction, roller bottles for large-scale cell culture, and scalable cell culture systems are increasingly used to facilitate manufacturing and support extended stability testing.
The design of the stability program must therefore account for:
- Material compatibility with plasticizers or adhesives.
- Oxygen or CO₂ diffusion through storage bags.
- Matrix component degradation, which may indirectly affect cell function or viability.
These aspects push cell therapy stability testing beyond the scope of ICH Q5C’s original intent, yet remain aligned with its spirit: ensuring quality, safety, and efficacy throughout shelf life.
5. Retest Period Not Applicable
ICH Q5C explicitly states that retesting is not appropriate for biological products due to their sensitivity to environmental and time-based degradation. This is even more critical in cell therapies, where:
- The product is administered as a single-use, patient-specific dose.
- Viability and potency may degrade rapidly and irreversibly.
“Re-analysis” or “re-release” is not feasible once the product’s use window has closed.
As such, the release and stability specifications must be absolutely reliable at the point of use, making real-time stability studies and stringent process controls indispensable.
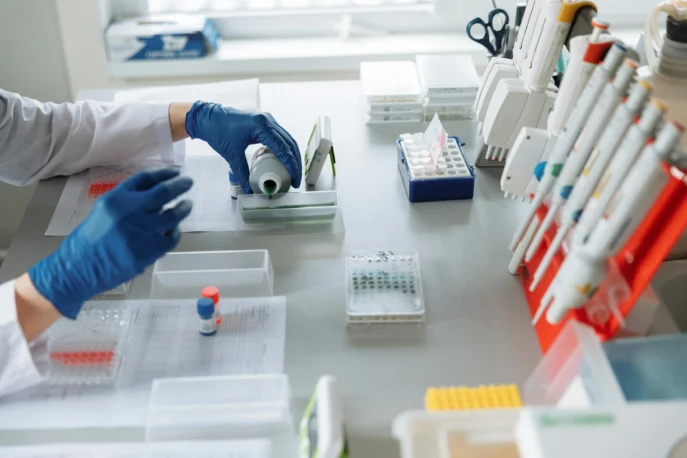
Regulatory Requirements for Stability
Under 21 CFR 211.166, stability testing is a regulatory requirement during all phases of product development under an IND. It ensures that the investigational product maintains acceptable chemical and physical characteristics for the duration of the proposed clinical study.
Key regulatory requirements include:
- A written stability testing program must be established to evaluate the product’s stability characteristics.
- Data from these studies should support decisions on storage conditions and expiration dating.
- Testing must be performed using reliable, meaningful, and specific methods, and in the same container-closure system intended for clinical use.
- Sample sizes and test intervals should be statistically justified.
- An adequate number of batches should be tested, and all data must be documented.
FDA Guidance on Stability Testing
FDA guidance emphasizes that stability analysis may include assessment of sterility, identity, purity, quality, potency, and activity. While the term “quality” lacks a precise regulatory definition, it generally refers to a product’s fitness for intended use, incorporating identity, strength, and purity.
Additional guidance points:
- At least one method in your stability program should be stability-indicating.
- The purpose of stability testing is to understand how product quality varies over time under different environmental conditions and to determine appropriate retest periods (for drug substances) or shelf lives (for drug products).
- A proposed stability profile should demonstrate the ability to detect changes in identity, potency, and purity.
Important caveat: These regulations and guidelines were developed primarily for small molecules and recombinant biologics—not for cell or gene therapy products, which require different considerations due to their complexity and sensitivity.
Importance of Stability-Indicating Test Methods
- FDA expects sponsors to incorporate stability-indicating methods in their testing programs. These analytical procedures must be able to detect meaningful changes in quality attributes over time, ensuring continued safety, potency, and quality under storage conditions.
- A stability-indicating method is defined as one that detects degradation or loss of product function.
- CGMP regulations allow flexibility in the choice of techniques but expect them to be scientifically justified.
For cell and gene therapies, the assessment of functional activity or potency is often considered a stability-indicating approach.
Ultimate Guide to Post-Thaw Viability & Functional Integrity in Cell Therapies
For cell therapy products, traditional chemical assays are often insufficient to reflect true product stability. Instead, functional and viability assays are essential to evaluate the biological integrity of the cells over time.
Post-thaw viability is a critical parameter for cell-based products, as delayed-onset cell death can occur hours or days after thawing. Understanding this phenomenon is key to improving the safety and efficacy of therapies.
Nonviable cells and debris may trigger unwanted immune or inflammatory responses.
Cell death can occur through multiple programmed and non-programmed mechanisms. For instance, autophagy may initially promote survival but can also have downstream effects such as genomic instability or loss of function.
Therefore, viability testing must go beyond simple live/dead counts. Results can vary depending on the assay, highlighting the importance of choosing assays appropriate to the specific cell system.
Stability-Indicating Assays for Cell Therapy Products
A wide range of analytical methods may serve as stability-indicating assays for cell therapies, depending on the product’s intended mechanism of action:
Key Feature | Method |
---|---|
Viability dyes | 7-AAD and Annexin V/PI, which detect apoptotic and dead cells. |
Flow cytometry | For evaluating surface markers and cell viability. |
Metabolic activity | Mitochondrial function assays |
Cell proliferation | CFSE labeling, Ki67 expression to measure proliferative capacity. |
Functional response | – Real-time calcium imaging, response to TNF-α or cytotoxic agents – Cytokine release assays to assess immune activation and functionality – Chromium-51 release or real-time imaging to evaluate target cell killing |
Why It Matters These assays are particularly valuable because they can detect subtle changes in function that might not be apparent through standard viability assays alone. In this way, they serve as functional indicators of stability, ensuring that the product maintains its intended biological activity throughout storage and use.
Container Selection and Its Impact on Cryopreservation and Stability
Why Container Choice Matters
The choice of cryopreservation container plays a critical role in the stability of cell therapy products, as it directly influences the physical and biological integrity of the cells during storage and handling. Stability testing, including both real-time and accelerated studies, evaluates how well the product maintains its viability, identity, and functionality over time under defined storage conditions.
Containers must not only be compatible with cryogenic temperatures but also provide a hermetic seal to prevent contamination and minimize thermal variability, both of which can compromise stability outcomes. For example, differences in wall thickness, thermal conductivity, and sealing methods between cryovials, cryobags, and other formats can lead to inconsistent cooling and thawing rates, affecting cell viability and function. Therefore, selecting an appropriate container is essential to ensure meaningful and reproducible stability data that supports product shelf-life, safety, and efficacy.
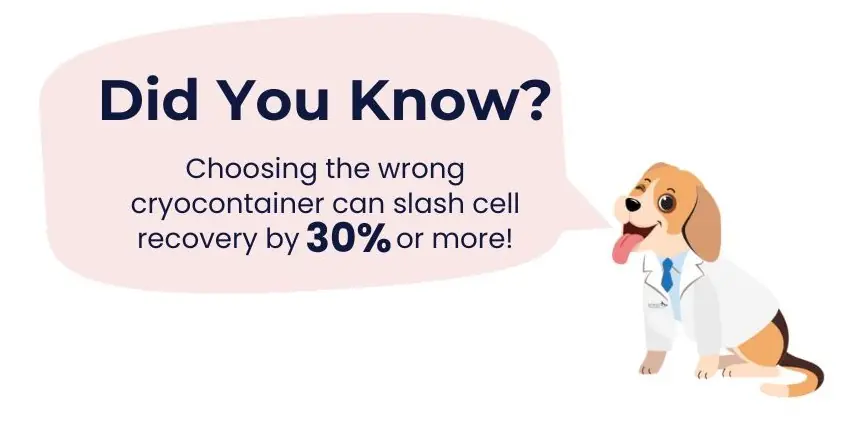
How to Select a Cryopreservation Containers
1. Alignment with Application:
Choice of container should match the intended use of the cell product.
- Research use: Small sterile cryovials (1–10 mL) are typically sufficient
- Therapeutic use: Requires hermetically sealed, medical-grade containers to prevent contamination
2. Contamination Risk:
- Unsealed containers may be exposed to contaminants from liquid nitrogen (LN) or introduced during the cryochain
- For parenteral administration, sealed containers are mandatory to meet sterility and safety standards
3. Cryovial Design Considerations:
- Medical cryovials often have thicker walls for structural integrity at ultra-low temperatures (106).
- May be made of materials with low thermal conductivity, affecting freezing rates.
- Use of dummy vials with temperature probes is recommended to verify alignment with programmed freezing protocols
- Especially important when QC samples are stored in vials while the therapeutic product is stored in cryobags.
4. Cryobag Use for Larger Volumes:
- Common for cell therapies needing >100 mL volumes.
- Cryobags are sterile, hermetically sealed, and typically overwrapped to prevent mechanical damage and maintain sterility (15).
5. Thermal Transfer Considerations:
- Trapped air between cryobag and overwrap acts as a thermal insulator—should be minimized.
- Metallic casings can be used to:
- Hold the bag flat, reducing risk of breakage at cryogenic temperatures.
- Improve heat transfer, ensuring a consistent thermal profile across the product.
- Promote uniform freezing and more reproducible post-thaw recovery.
Examples of Suitable Cryocontainers
Container Type | Volume Range | Key Features | Suitability |
---|---|---|---|
Medical-grade cryovials | 0.5–50 mL | Complies with medical device standards | Clinical use only; standard lab cryovials not acceptable |
Cryobags | 10 mL to >100 mL | Sterile, sealed, often overwrapped | Large-volume cell therapies; not ideal for <5 mL |
Cryo straws | ≤0.5 mL | Efficient heat-sealing | Originally for reproductive cells; now used in vitrified cell therapies |
Cryopreservable syringes | In development | Allows direct post-thaw administration | Expected future clinical use |
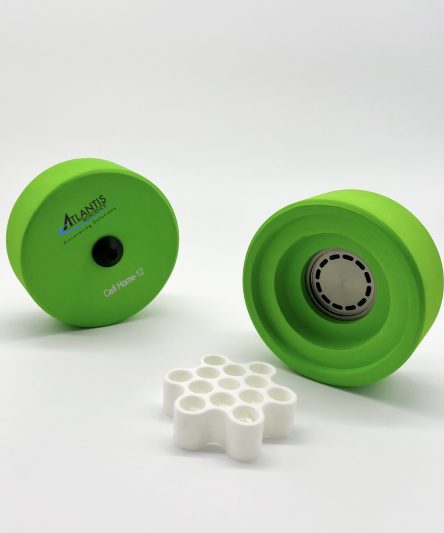
Types of Stability Studies
As with any preservation method, it’s important to demonstrate that the characteristics of the cells remain consistent before and after preservation to ensure the cell product maintains its intended potency and efficacy. Since preservation and recovery involve dynamic changes in cell condition, relying solely on a single quality control check immediately after thawing can be misleading. Therefore, when developing new or modified preservation techniques, it was determined that cell recovery should be tracked over time after thawing, to build a profile of how the cells recover and replicate until they regain full functionality.
1. Real-Time Stability Studies
Purpose: To determine the actual shelf life of a drug product or substance under recommended storage conditions.
Key Aspect | Details |
---|---|
Methodology | Store under label-claimed storage conditions (e.g., 2–8°C for liquid biologics, ≤–150°C for cryopreserved cell therapies), and tested at predetermined intervals (e.g., 0, 3, 6, 12, 18, 24 months). |
Parameters Monitored | Biologics: Aggregation, potency (bioassay), purity, pH, appearance, subvisible particles, glycosylation. Cell Therapy: Viability, phenotype (e.g., % CD3+, % CD8+), sterility, functionality (e.g., cytokine release or cytotoxicity assays) |
Significance | Mandatory for determining the product’s true shelf life and for regulatory submissions. It must reflect the final container-closure system, final formulation, and intended use/storage. |
2. Accelerated Stability Studies
Purpose: To estimate product stability and shelf life by subjecting the product to elevated stress conditions over a shorter time frame than real-time studies.
Key Aspect | Details |
---|---|
Methodology | Store at higher-than-recommended temperatures (e.g., 25°C or 40°C) for predetermined durations (e.g., 1 week to 6 months). The resulting data may be modeled (e.g., using the Arrhenius equation) to predict degradation kinetics and extrapolate long-term stability. |
Parameters Monitored | The same quality attributes assessed in real-time studies are monitored, but at increased frequency to detect early signs of degradation. |
Significance | Accelerated testing is especially valuable during early-stage development or when rapid shelf-life estimation is needed to support clinical trial supply planning or conditional regulatory approval. |
Example: A manufacturer’s master cell bank (MCB) may be intended for use over several decades. Accelerated stability studies provide a faster means of evaluating long-term stability by exposing MCB aliquots to elevated temperatures (e.g., -80°C, -20°C, or even +5°C for short periods). These stress tests help assess the robustness of the preservation method (e.g., cryopreservation) and the potential for degradation in key attributes such as cell viability, phenotype, and functionality, particularly in the event of temperature excursions during storage, transport, or handling.
3. Forced Degradation Studies
Purpose: To intentionally degrade the product under extreme stress conditions to:
- Inform formulation development and packaging decisions
- Identify degradation pathways
- Develop stability-indicating analytical methods
Stress Condition | Methodology |
---|---|
Heat | 60°C for 24–72 hours |
pH Stress | pH 2 or pH 10 exposure |
Oxidation | H₂O₂ or light exposure |
Freeze/Thaw | Repeated cycles (critical for cell therapies) |
Significance: Not used to set shelf life, but critical in early-stage development and regulatory filings. It helps establish degradation markers and ensures analytical methods can detect these.
Example: A lentiviral vector exposed to multiple freeze/thaw cycles shows a drop in infectivity; this leads to a decision to minimize processing steps post-thaw.
4. Long-Term Stability Studies
Purpose: To verify product stability under intended long-term storage and provide a comprehensive shelf-life claim for commercial approval.
Key Aspect | Details |
---|---|
Methodology | Store under real-use conditions (e.g., 2–8°C, -20°C, -80°C, or ≤–150°C) and tested over an extended timeline (e.g., 24–36 months). May include a “bridging” study to support changes in formulation or packaging. |
Significance | Core data for regulatory filings (drug substance, fThis is the core data package required for regulatory approval of biologics. Often, multiple studies are conducted:
|
Example: A cryopreserved CAR-T cell therapy is stored in vapor-phase liquid nitrogen and tested at 0, 3, 6, 12, 18, and 24 months for viability, transduction efficiency, and killing activity. Data support the 24-month shelf life.
5. In-Use Stability Studies
Purpose: To assess the stability of the product after preparation for administration, such as:
- During dosing or infusion
- Post-thaw
- After dilution or reconstitution
Key Aspect | Details |
---|---|
Methodology | Thaw/prepare as per clinical instructions and kept at room temperature or 2–8°C for a defined period (e.g., 4 to 24 hours). Samples are tested for changes in quality attributes. |
Significance | Critical for clinical use where infusion may be delayed or drugs must be handled at bedside. |
Example: Post-thaw stability of a T cell product is evaluated for 6 hours at 20–25°C. Viability and cytotoxic function are measured hourly. Data support the claim that the thawed product must be infused within 4 hours.
6. Shipping & Excursion Studies
Purpose: To evaluate the product’s stability when exposed to transient temperature excursions during transport (e.g., if cold chain fails).
Key Aspect | Details |
---|---|
Methodology | Simulated shipping studies use temperature-controlled chambers or actual transport routes (e.g., local or overseas shipping). Temperatures are monitored, and product is tested before and after. |
Significance | Essential for distribution planning, ensuring product remains within specifications even with minor transport deviations. |
Example: A plasmid used for ex vivo gene editing is tested for a 48-hour shipment at 2–8°C, including a 6-hour excursion to 15°C. Results confirm no impact on supercoiled content or transfection efficiency.
Conclusion: A New Paradigm for Cell Therapy Stability
Stability testing for cell therapy products requires a paradigm shift from traditional pharmaceutical approaches toward biologically meaningful, product-specific strategies. As cell viability and function can be profoundly impacted by storage conditions, thawing processes, and delayed-onset cell death, it is no longer sufficient to rely solely on conventional metrics such as simple viability counts. Instead, comprehensive assessments that integrate cell phenotype, metabolic activity, functional responsiveness, and death kinetics are essential to ensuring the safety, potency, and overall quality of the final product.
Establishing stability-indicating methods tailored to the unique characteristics of each cell therapy product will enhance regulatory compliance, support consistent clinical performance, and ultimately improve patient outcomes. As the field evolves, a deeper understanding of cellular biology combined with innovative analytical techniques will be critical to advancing robust, reliable, and effective cell-based therapies.
The future of cell therapy stability lies in measuring what truly matters: not just whether cells live, but how they function.
References
- Stacey GN et al. Preservation and stability of cell therapy products: recommendations from an expert workshop. Regen Med. 2017;12(5):611–622.
- Meneghel Jet al. Cryopreservation as a key element in the successful delivery of cell-based therapies—a review. Front Med (Lausanne). 2020;7:592242.
- François M et al. Cryopreserved mesenchymal stromal cells display impaired immunosuppressive properties as a result of heat-shock response and impaired interferon-γ licensing. Cytotherapy. 2012;14(2):147–152.
- U.S. Food and Drug Administration. 21 CFR 211.166 – Stability testing.
- International Council for Harmonisation of Technical Requirements for Pharmaceuticals for Human Use (ICH).
- Q5C: Quality of Biotechnological Products: Stability Testing of Biotechnological/Biological Products (July 1996).
- Q1A(R2): Stability Testing of New Drug Substances and Products (November 2003).
- Q1E: Evaluation of Stability Data (June 2004).