Table of Contents
- Current Cryopreservation Strategies in Cell and Gene Therapy
- The Role of DMSO in Cryopreservation: Balancing Efficacy with Toxicity
- Why Proteins Are Used in Cryopreservation
- Best‑Practice Checklist for Adopting Defined, Xeno‑Free Media
- CryoEase-PF: A Safer, Defined Alternative for Sensitive Cell Preservation
Cryopreservation is a cornerstone technique in cell and gene therapy research, enabling the long-term storage and transport of biological material while aiming to preserve viability and functionality. For sensitive cell types—such as mesenchymal stem cells (MSCs), CAR-T cells, and induced pluripotent stem cells (iPSCs)—this process is particularly high-stakes, where every component of the freezing medium can make or break therapeutic success.
To mitigate the damaging effects of ice crystal formation, cryoprotective agents like dimethyl sulfoxide (DMSO) and proteins, often in the form of fetal bovine serum (FBS) or human serum albumin (HSA), are commonly used. However, these additives introduce significant risks if not carefully managed, especially for cells destined for clinical applications.
In this blog, we explore the hidden pitfalls of DMSO and protein in cryopreservation media—from cytotoxicity and batch variability to immunogenic concerns—and why researchers must approach these components with caution. A thorough understanding of these risks is critical to developing optimised cryopreservation strategies that ensure the reliability, safety, and therapeutic potential of cell and gene therapies.
Current Cryopreservation Strategies in Cell and Gene Therapy
The success of cell and gene therapy hinges on the ability to preserve cellular products without compromising their viability, functionality, or therapeutic potential. Cryopreservation—the process of cooling cells to ultra-low temperatures (typically around -80°C or -196°C using liquid nitrogen)—halts metabolic activity, enabling long-term storage and transport. However, the freezing and thawing process itself poses significant challenges, including ice crystal formation, osmotic stress, and oxidative damage, all of which can impair cell survival and performance.
To mitigate these risks, optimised cryopreservation protocols must balance cooling rates, storage conditions, and the use of cryoprotective agents (CPAs). Two primary approaches are commonly employed:
Slow Freezing
A widely used method that cools cells at a controlled rate of approximately −1°C per minute. This rate allows water to exit the cytoplasm, which is then partially replaced by CPAs like DMSO. The purpose is to minimise intracellular ice formation, which can cause membrane rupture and loss of viability.
The cooling rate is carefully matched to the permeability of the cell membrane, ensuring sufficient time for water efflux and CPA uptake before ice crystals can form. Slow freezing is typically performed using controlled-rate freezers or freezing containers.
This technique is favoured for its low contamination risk, minimal hands-on manipulation, and broad applicability across cell types—including those used in cell and gene therapy research. It also offers consistent outcomes without requiring specialised cryopreservation expertise.
Vitrification
An ultra-rapid cooling technique in which aqueous cell suspensions are exposed directly to liquid nitrogen, causing the solution to transition instantly into a glass-like, ice-free state. This method avoids the formation of ice crystals, which can damage cell structures, making vitrification especially effective for highly sensitive cells such as oocytes and certain stem cells.
Prior to cooling, cells or tissues are exposed to high concentrations of CPAs—typically 40–60% (w/v)—to prevent ice nucleation. To be successful, vitrification requires extremely fast cooling and warming rates, along with careful control of variables such as sample volume, viscosity, and the precise formulation of CPA mixtures.
While vitrification offers the major advantage of minimising freeze damage and preserving high post-thaw viability, it presents several challenges. These include CPA toxicity, the need for precise manipulation skills, and a non-negligible risk of microbial contamination—especially in open systems. As such, vitrification is best suited for applications where maintaining cellular integrity is critical, and where technical expertise and stringent aseptic conditions can be ensured.
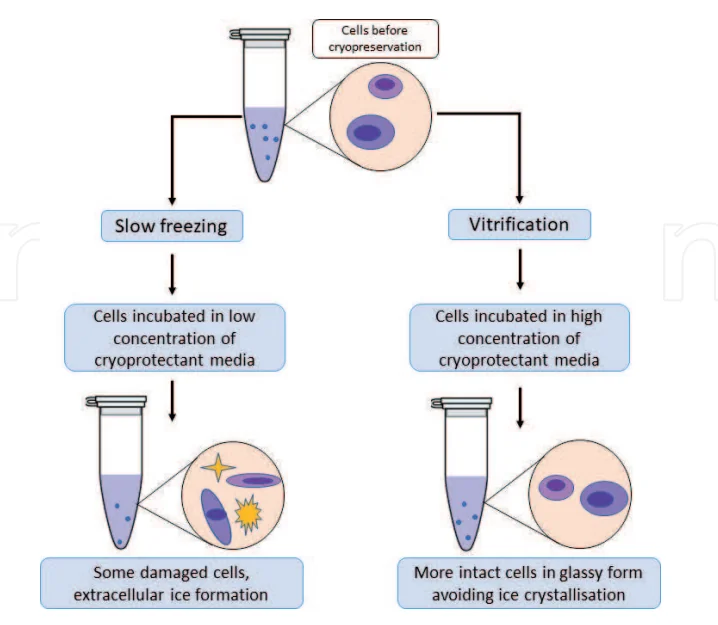
Reference: DOI: 10.5772/intechopen.101750
Despite these advancements, the choice of CPAs remains a critical variable. Proteins (e.g., serum albumin) and small-molecule additives like DMSO are widely used but come with trade-offs in safety, consistency, and post-thaw recovery. The following sections explore the advantages, limitations, and risks associated with these key components in cell and gene therapy applications.
The Role of DMSO in Cryopreservation: Balancing Efficacy with Toxicity
DMSO has been the cornerstone of cryopreservation since its introduction over the past 60 years, revolutionising the long-term storage of living cells. As the most widely used penetrating CPA, it is indispensable for preserving sensitive cell types. However, despite its effectiveness, DMSO presents significant challenges—ranging from cellular toxicity to clinical side effects—that have prompted researchers to seek safer alternatives.
Why DMSO is the Gold Standard in Cryopreservation
DMSO has long been considered the gold standard CPA due to its unique ability to penetrate cell membranes and prevent intracellular ice formation, which is a primary cause of cell damage during freezing.
Key Reasons for Its Dominance:
- Membrane Permeability: DMSO rapidly enters cells and equilibrates with the intracellular environment. This prevents ice crystals from forming inside the cell by reducing the amount of freezable water.
- Effective at Low Concentrations: Typically used at 5–10% (v/v), DMSO offers excellent protection without requiring excessively high concentrations that would increase toxicity.
- Broad Applicability: DMSO is compatible with a wide range of cell types—including hematopoietic stem cells, MSCs, lymphocytes, and various primary and immortalised cell lines—making it a versatile tool across research and clinical applications.
- Clinically Validated: DMSO has a long-standing track record in clinical cryopreservation protocols, including hematopoietic stem cell transplants. Its efficacy and safety profile are well-documented in regulatory frameworks.
- Cost-Effectiveness and Availability: It is widely accessible, stable at room temperature, and economical, making it a practical choice for both research labs and commercial biobanks.
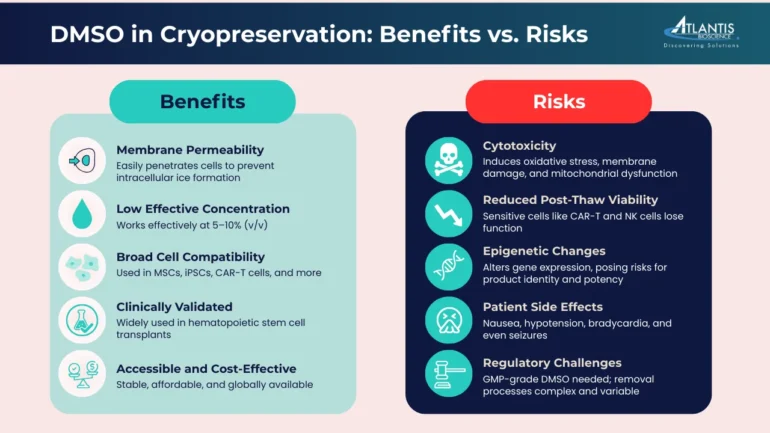
Key Limitations and Risks of DMSO
Despite its widespread use in research and clinical cryopreservation, DMSO is not without significant risks. Its cytotoxic nature, potential to impair cellular functions, and clinical side effects raise serious concerns about its long-term suitability—especially in cell and gene therapy applications, where product integrity and patient safety are paramount.
1. Cytotoxicity and Functional Impairment
While DMSO is effective in preventing intracellular ice formation, it is inherently toxic to cells, even at concentrations as low as 5–10% for some sensitive cell types.
- Disruption of Cellular Metabolism: DMSO can compromise mitochondrial respiration, induce oxidative stress, and damage cell membranes. These effects are particularly pronounced during the thawing phase, when cells are most vulnerable.
- Loss of Post-Thaw Viability and Function: Certain immune cells—such as natural killer cells, dendritic cells, and CAR-T cells—are highly sensitive to DMSO exposure. Post-thaw, these cells may show reduced viability, proliferative capacity, and effector functions, limiting their therapeutic utility.
- Epigenetic and Transcriptional Perturbations: Extended DMSO exposure has been associated with changes in gene expression and epigenetic modifications, which may compromise the potency, identity, and stability of therapeutic cell products. These effects can introduce variability into manufacturing and raise concerns for regulatory compliance.
2. Clinical Side Effects in Patients
Infusion of DMSO-containing cell products can result in adverse reactions in patients, particularly when large volumes or high concentrations are involved.
- Acute Infusion-Related Reactions: Common side effects include nausea, vomiting, abdominal cramps, hypotension or hypertension, and in some cases, anaphylaxis-like responses
- Cardiovascular and Neurological Events: In rare but serious cases, DMSO infusion has been linked to bradycardia, seizures, and encephalopathy—especially in pediatric or immunocompromised patients
- Hemolysis Risk: Residual DMSO can cause membrane instability in red blood cells, potentially leading to hemolysis if red cell components are included in the infusion or not thoroughly washed post-thaw
3. Regulatory and Manufacturing Challenges
DMSO introduces several challenges that complicate the scale-up and clinical translation of cell-based therapies:
- Clinical-Grade DMSO Requirements: When used in cell therapy manufacturing, DMSO must meet stringent quality standards (e.g., USP or Ph. Eur. grade), and its handling requires careful documentation to meet regulatory compliance for Good Manufacturing Practice (GMP) environments.
- Inconsistency Between Suppliers: The purity and performance of DMSO can vary between manufacturers, which may impact the reproducibility of cryopreservation protocols. This inconsistency becomes critical when scaling up processes for regulatory filings or clinical-grade production.
- Downstream Processing Burden: Removing residual DMSO post-thaw—typically via dilution or washing—adds time and complexity. These steps can lead to significant cell loss, functional changes, or activation-induced apoptosis, which ultimately reduces yield and product consistency.
- Industry Trend Toward DMSO Minimisation: Although not mandated, many developers are proactively reducing DMSO concentrations or exploring DMSO-free alternatives to align with evolving expectations for safer, more defined cell therapy products.
Why Proteins Are Used in Cryopreservation
Proteins—particularly serum-derived proteins like albumin and FBS—play several critical roles in traditional cryopreservation protocols. Their inclusion helps mitigate cellular damage from the mechanical, osmotic, and oxidative stresses induced by freezing and thawing processes.
1. Membrane Stabilisation and Ice Recrystallisation Inhibition
During cryopreservation, cells are vulnerable to ice crystal-induced mechanical damage, particularly during thawing when recrystallisation can amplify injury. Proteins help in several ways:
- Surface Protection: Proteins like albumin adsorb onto the cell membrane, forming a hydrated protective layer that minimises direct contact with ice crystals. This cushioning effect reduces membrane rupture and mechanical stress
- Ice Recrystallisation Inhibition: Some proteins mimic the action of antifreeze proteins found in nature, helping to modulate ice crystal growth and inhibit recrystallisation, which is critical for preserving delicate cell types such as stem cells and immune cells.
- Colloidal Stability: Albumin and other proteins act as colloidal stabilisers, preventing cell aggregation or clumping during both the freezing and thawing stages. This improves uniformity in cell suspensions and facilitates easier downstream processing.
2. Osmotic and Oxidative Stress Mitigation
The freeze-thaw cycle imposes significant osmotic shifts and oxidative stress, both of which can compromise cell viability.
- Osmoprotection: Proteins help buffer osmotic imbalances by modulating water movement and solute concentrations across the membrane. This reduces excessive cell shrinkage or swelling, which can otherwise cause irreversible membrane damage.
- Antioxidant Capacity: Serum-based cryopreservatives like FBS naturally contain antioxidants, including glutathione and catalase, which help neutralise reactive oxygen species (ROS) generated during thawing. This antioxidant buffering limits oxidative damage to cellular components and prevents apoptotic cascades triggered by stress.
3. Growth Factors and Post-Thaw Recovery Support
Beyond immediate protection during cryopreservation, proteins contribute to post-thaw recovery, especially for sensitive or metabolically active cells:
- Biological Activity: FBS is rich in growth factors, hormones, cytokines, lipids, and vitamins. These molecules support cell metabolism, proliferation, and repair mechanisms after thawing.
- Stem Cell Support: In the case of MSCs, hematopoietic stem cells (HSCs), and primary cells, the complex milieu in FBS can enhance recovery and reattachment, accelerating the return to normal function.
Protein Additives – Not Always Harmless
While proteins such as FBS, HSA, or plasma derivatives are widely used in cryopreservation for their protective effects, they come with significant risks—especially when developing clinical-grade or reproducible research products. These risks are particularly concerning in the context of cell and gene therapy, where product consistency, purity, and safety are paramount.
1. Batch-to-Batch Variability
One of the most pressing issues with biologically derived proteins—especially FBS—is lot-to-lot variability. Even when sourced from the same supplier, different batches may vary in protein content, osmolarity, or presence of undefined factors. This variability can lead to:
- Unpredictable cryopreservation outcomes, including inconsistent cell viability and recovery.
- Difficulty in scaling up or transferring protocols from research to GMP settings.
- Challenges in process validation, a critical step for regulatory approval.
2. Immunogenicity and Contaminant Risks
Proteins sourced from animals or pooled human plasma may introduce unwanted xeno- or allo-antigens, which can trigger immune responses in patients—especially in allogeneic cell therapies.
Moreover, these protein supplements carry the risk of biological contaminants, including:
- Endotoxins that can trigger cytokine storms.
- Prions or viral particles that evade filtration.
- Unknown adventitious agents that raise red flags during regulatory inspection.
Even “clinical-grade” human albumin may not be fully risk-free. The presence of these contaminants complicates both GMP manufacturing and regulatory clearance for clinical applications.
3. Interference with Downstream Applications
In addition to safety concerns, protein additives can complicate downstream processes, especially in gene therapy and EV research:
- During transduction or electroporation, proteins can inhibit viral entry, reduce transfection efficiency, or promote aggregation.
- In extracellular vesicle (EV) studies, serum-derived proteins may contaminate EV preparations, leading to false-positive results or compromised biomarker analysis.
- In proteomic or omics workflows, residual proteins may introduce background noise, masking critical signals or introducing artifacts.
Moving Toward Defined, Xeno‑Free Alternatives
The safety, regulatory, and supply‑chain pressures outlined above have accelerated a shift away from “classic” FBS + 10 % DMSO recipes toward chemically‑defined, xeno‑free cryopreservation systems. Many commercial manufacturers have developed next-generation media that replace serum and other animal-derived components with recombinant or synthetic stabilisers—and, in many cases, significantly reduce or eliminate DMSO altogether.
Best‑Practice Checklist for Adopting Defined, Xeno‑Free Media
✓ Match DMSO Level to Application
- Prefer 0 % DMSO for autologous infusions or very sensitive cells (e.g., MSCs, iPSCs, NK cells).
- For products where some DMSO is still advantageous, cap at ≤ 5 % and plan a rapid, closed‑system wash step.
✓ Validate per Cell Type & Indication
- Run pilot freeze–thaw studies measuring viability, phenotype (flow cytometry), potency/read‑outs (e.g., CFU‑F for MSCs, cytotoxicity for CAR‑T).
- Confirm functional recovery, not just membrane integrity.
✓ Use Controlled‑Rate Freezing and Closed Systems
- Maintain ~1 °C · min⁻¹ cool‑down (slow‑freeze) unless the product requires vitrification.
- Employ closed‑bag or vial formats to avoid microbial ingress and preserve GMP chain‑of‑identity.
✓ Shorten Post‑Thaw CPA Exposure
Warm quickly (30–60 s in 37 °C water bath), dilute CPA within ≤ 10 min, and complete washes in a BSC to minimise cytotoxic effects—even with “low‑tox” CPAs.
CryoEase-PF: A Safer, Defined Alternative for Sensitive Cell Preservation
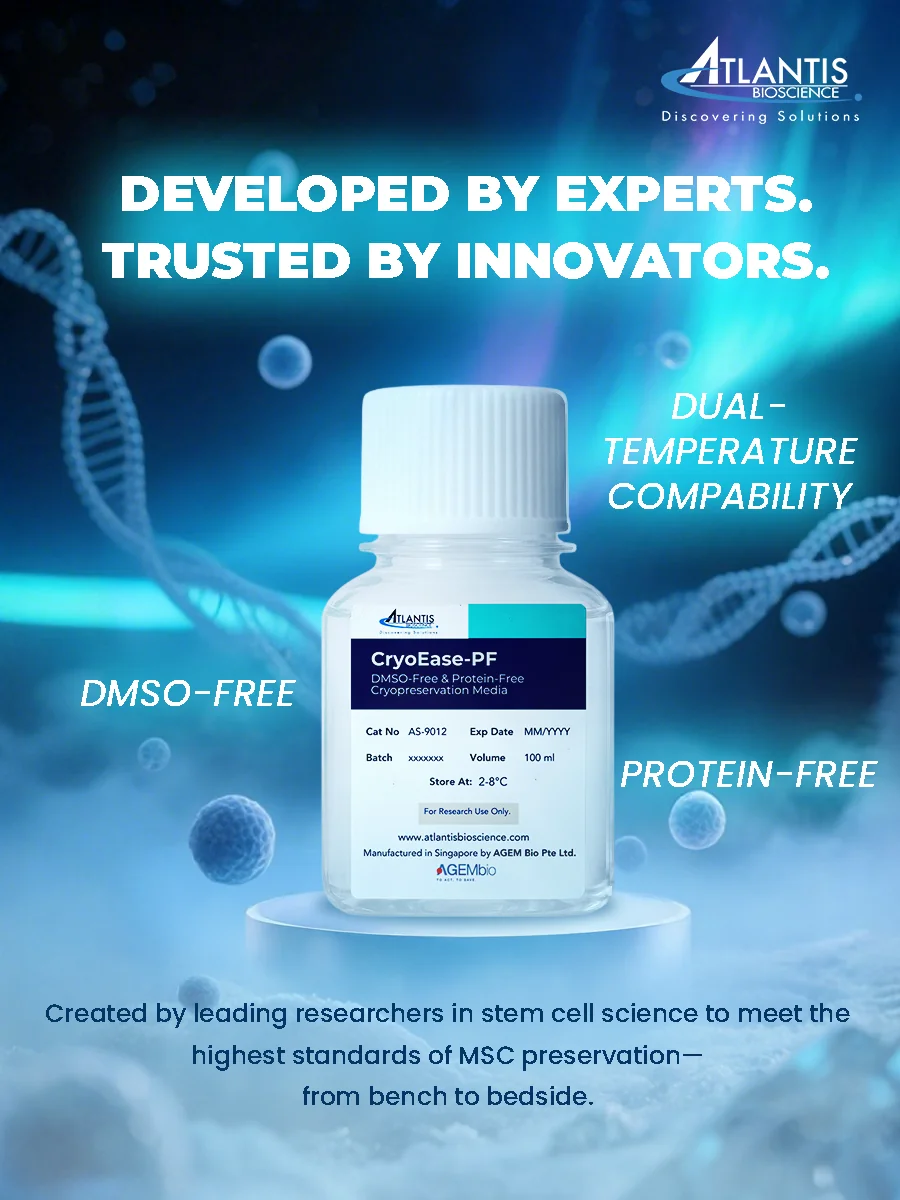
CryoEase-PF, a newly launched cell cryopreservation media by Atlantis Bioscience, addresses the challenges associated with traditional cryopreservation agents like DMSO and serum proteins. This chemically defined, xeno-free solution is specifically formulated for the preservation and transport of sensitive cells, including MSCs.
Key Advantages:
- DMSO-Free and Protein-Free Composition: By eliminating DMSO and animal-derived proteins, CryoEase-PF reduces cytotoxicity and variability, ensuring higher post-thaw cell viability and functional integrity.
- Dual-Temperature Compatibility: CryoEase-PF is effective at both subzero (−80°C) and hypothermic (4–10°C) temperatures, providing flexibility for various storage and transport conditions.
- Enhanced Cell Viability: Studies have demonstrated that MSCs preserved with CryoEase-PF retain over 85% viability after 72 hours at 4°C, outperforming other formulations that show less than 75% viability under similar conditions.
- Maintained Functional Integrity: Post-thaw, MSCs exhibit consistent population doubling times and retain their trilineage differentiation potential, aligning with the criteria set by the International Society for Cell and Gene Therapy (ISCT).
- GMP-Compliant Materials: Formulated with GMP-compliant materials, CryoEase-PF supports a seamless transition from research to clinical applications, even across international borders.
CryoEase-PF’s innovative formulation simplifies logistics by reducing reliance on complex cold chain systems, making it ideal for nationwide and international shipping of sensitive cells. Its compatibility with both short-term hypothermic storage and long-term cryopreservation offers researchers and clinicians a versatile solution for maintaining cell quality during transport and storage.
References:
- Aljaser, Feda.Cryopreservation Methods and Frontiers in the Art of Freezing Life in Animal Models. 2022 10.5772/intechopen.101750. DOI: 10.5772/intechopen.101750
- Amini M, Benson JD. Technologies for Vitrification Based Cryopreservation. Bioengineering (Basel). 2023 Apr 23;10(5):508. doi: 10.3390/bioengineering10050508.
- Awan M, Buriak I, Fleck R, Fuller B, Goltsev A, Kerby J, Lowdell M, Mericka P, Petrenko A, Petrenko Y, Rogulska O, Stolzing A, Stacey GN. Dimethyl sulfoxide: a central player since the dawn of cryobiology, is efficacy balanced by toxicity? Regen Med. 2020 Mar;15(3):1463-1491. doi: 10.2217/rme-2019-0145. Epub 2020 Apr 28.
- Ekpo MD, Boafo GF, Xie J, Liu X, Chen C, Tan S. Strategies in developing dimethyl sulfoxide (DMSO)-free cryopreservation protocols for biotherapeutics. Front Immunol. 2022 Oct 5;13:1030965. doi: 10.3389/fimmu.2022.1030965.
- Erol OD, Pervin B, Seker ME, Aerts-Kaya F. Effects of storage media, supplements and cryopreservation methods on quality of stem cells. World J Stem Cells. 2021 Sep 26;13(9):1197-1214. doi: 10.4252/wjsc.v13.i9.1197.
- Fujita Y, Nishimura M, Komori N, Sawamoto O, Kaneda S. Protein-free solution containing trehalose and dextran 40 for cryopreservation of human adipose tissue-derived mesenchymal stromal cells. Cryobiology. 2021 Jun;100:46-57. doi: 10.1016/j.cryobiol.2021.03.011. Epub 2021 Apr 3.
- Jaiswal AN, Vagga A. Cryopreservation: A Review Article. Cureus. 2022 Nov 16;14(11):e31564. doi: 10.7759/cureus.31564.
- Joules A, Mallya AS, Louwagie T, Yu G, Hubel A. Probing mechanisms of cryopreservation damage to natural killer cells. Cytotherapy. 2025 Jan 25:S1465-3249(25)00035-0. doi: 10.1016/j.jcyt.2025.01.012.
- Weng L. Cell Therapy Drug Product Development: Technical Considerations and Challenges. J Pharm Sci. 2023 Oct;112(10):2615-2620. doi: 10.1016/j.xphs.2023.08.001. Epub 2023 Aug 6.
- Whaley D, Damyar K, Witek RP, Mendoza A, Alexander M, Lakey JR. Cryopreservation: An Overview of Principles and Cell-Specific Considerations. Cell Transplant. 2021 Jan-Dec;30:963689721999617. doi: 10.1177/0963689721999617.
- Windrum P, Morris TC, Drake MB, Niederwieser D, Ruutu T; EBMT Chronic Leukaemia Working Party Complications Subcommittee. Variation in dimethyl sulfoxide use in stem cell transplantation: a survey of EBMT centres. Bone Marrow Transplant. 2005 Oct;36(7):601-3. doi: 10.1038/sj.bmt.1705100. PMID: 16044141.