Summary
MSCs can be derived from umbilical cord (UC-MSCs), bone marrow (BM-MSCs), and adipose tissue (AD-MSCs). BM-MSCs has traditionally served as the primary stem cell source for an extended period, however, the challenges and risks associated with their procurement have sparked investigations into alternative and more convenient sources. MSCs play an important role in cell therapy research and have been shown to demonstrate clinical applications in treating different diseases. However, before applying MSCs from any source, thorough evaluations of their safety and efficacy are imperative. This evaluation should commence with animal models and progress to clinical trials, ensuring a comprehensive understanding of their potential benefits and risks.
Mesenchymal stem cells (MSCs) have garnered significant attention in the field of regenerative medicine due to their unique properties and potential therapeutic applications. These multipotent cells can differentiate into various cell types, contribute to tissue repair, and modulate the immune system. What makes MSCs even more intriguing is their diverse range of sources, each exhibiting distinct functional capacities. In this blog, we will delve into the different MSCs sources and their varied potentials.
Bone Marrow-Derived MSCs
Discovered by Friedenstein in 1976, Bone Marrow-Derived MSCs (BM-MSCs) have since emerged as a crucial player in regenerative medicine. Although bone marrow stands out as a primary source of human MSCs, the quantity of MSCs within the bone marrow is surprisingly low, constituting only 0.001–0.01% of the total stromal cell population, despite its richness in hematopoietic stem cells. Therefore, ex vivo expansion is essential to achieve the clinically required cell quantity.
Complicating matters, the quantity and quality of BM-MSCs decline with age, posing a significant hurdle for their application in older patients. The challenge lies in expanding autologous BM-MSCs efficiently to therapeutic levels. Furthermore, the collection of BM-MSCs is an invasive and painful procedure requiring general anesthesia, limiting their availability for therapeutic applications.
However, despite these challenges, both preclinical and clinical trials have shown promising outcomes for BM-MSCs in treating various diseases, with minimal adverse effects during follow-up periods. Notably, BM-MSCs have exhibited effectiveness in treating conditions such as osteoarthritis, neurodegenerative diseases, sports-related injuries, and graft-versus-host disease (GvHD) following organ transplantation.
Adipose Tissue-Derived MSCs
Adipose tissue-derived MSCs (AD-MSCs) present a promising alternative to BM-MSCs. It is obtained from subcutaneous adipose tissue through a liposuction procedure, AD-MSCs can be rapidly acquired in substantial numbers with high cellular activity, making them a more accessible resource.
Compared to BM-MSCs, the isolation of AD-MSCs is less invasive, more convenient, and easier. AD-MSCs are a significant component of the stromal vascular fraction (SVF), constituting approximately 50% of the cells within this fraction – which is almost 500-fold greater than the expression of BM-MSCs within their niche. AD-MSCs may be used clinically without the need for extensive expansion if a sufficiently large volume of lipoaspirate is harvested. Like BM-MSCs, the quantity and quality of AD-MSCs decline with age. However, AD-MSCs closely resemble BM-MSCs morphologically, phenotypically, and functionally, making them an attractive alternative.
Different regions of white adipose tissue – subcutaneous adipose tissue (SAT) and visceral adipose tissue (VAT) – yield MSCs with distinct genetic profiles, secretomes, and stem cell markers due to their varied developmental origins and microenvironments.
AD-MSCs boast stability in long-term cell cultures, effective in vitro expansion, and high multilineage differentiation potential. They maintain the ability to differentiate into cells of mesodermal origin, exhibiting low immunogenicity and powerful immunomodulatory effects. With less than 1% expressing the HLA-DR protein, AD-MSCs demonstrate immunosuppressive effects, making them suitable for allogeneic transplantation and therapies for resistant immune disorders.
Additionally, AD-MSCs surpass BM-MSCs in immunomodulatory effects, attributed to their elevated cytokine secretion levels. Their higher proliferative capability and lower senescence ratio, coupled with retained differentiation potential, make AD-MSCs genetically and morphologically stable in long-term culture. Although AD-MSCs exhibit a lower differentiation potential to osteogenic and chondrogenic lineages compared to BM-MSCs, AD-MSCs are predisposed toward adipocyte lineage differentiation. AD-MSCs secrete pro-angiogenic factors like Vascular Endothelial Growth Factor (VEGF) and Platelet-Derived Growth Factor (PDGF), making them promising candidates for conditions such as myocardial infarction.
Widely recognised for their versatility, AD-MSCs present a viable solution with broad therapeutic, including autoimmune diseases, cardiovascular diseases, skin diseases, and orthopedic diseases.
Umbilical Cord-Derived MSCs
Umbilical Cord-Derived MSCs (UC-MSCs) can be derived from various sources, such as Wharton’s Jelly, cord lining, and the peri-vascular region of the umbilical cord. The umbilical cord, often discarded as medical waste, serves as an abundant and non-invasively obtainable reservoir of MSCs. The extraction process of UC-MSCs is non-invasive, eliminating the need for obtaining cells directly from the patient. MSCs are sourced ethically from donated human umbilical cords.
In comparison to BM-MSCs and AD-MSCs, UC-MSCs exhibit a higher proliferative potential, enabling more efficient in vitro expansion and yielding higher cell numbers. These multipotent cells can differentiate into various cell types, including those derived from ectoderm and mesoderm. UC-MSCs express pluripotency gene markers Oct-4, nanog, and Sox-2 at a level lower than in embryonic stem cells (ESCs) but higher than in BM-MSCs. This suggests that UC-MSCs may represent a subset of primitive stem cells.
Similar to BM-MSCs and AD-MSCs, UC-MSCs secrete growth factors, cytokines, and chemokines, enhancing cellular repair mechanisms. These functions contribute to the anti-inflammatory and immunomodulatory properties characteristic of MSCs. Like AD-MSCs, UC MSCs expressed similar markers to BM-MSCs, but have low expression of HLA-DR reducing the risk of transplantation rejection, and facilitating their use in allografts.
UC-MSCs have been tested in multiple clinical trials for different indications, including diabetes type I, SLE, multiple sclerosis, rheumatoid arthritis, Crohn’s disease, allograft-related diseases, and infections, i.e., HIV and sepsis.
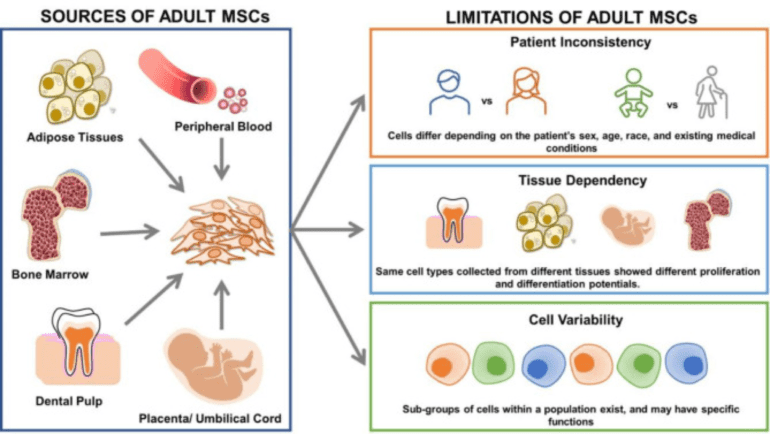
Credit: Prakash N, Kim J, et.al., doi: 10.1186/s40824-023-00371-0.
Reproduced under the Creative Commons license
Table 1: Comparison between MSCs isolated from different sources.
Characteristics | BM-MSC | AD-MSC | UC-MSC |
Harvesting Procedure | Invasive | Minimally Invasive | Non-Invasive |
Cell Yield | Lower | Higher | Lower |
Effect of age on cell quantity and quality | Declines with age | Declines with age | Unaffected |
Proliferative Capacity | Lower proliferative potential | Lower proliferative potential | Higher proliferative potential |
Expression of embryonic markers | Lower | Lower | Higher |
Immunomodulatory properties | Good | Good | Good |
References:
Álvaro-Gracia JM, Jover JA, García-Vicuña R, et al. Intravenous administration of expanded allogeneic adipose-derived mesenchymal stem cells in refractory rheumatoid arthritis (Cx611): results of a multicentre, dose escalation, randomised, single-blind, placebo-controlled phase Ib/IIa clinical trial. Ann Rheum Dis. 2017;76(1):196-202. doi:10.1136/annrheumdis-2015-208918
Berebichez-Fridman R, Montero-Olvera PR. Sources and Clinical Applications of Mesenchymal Stem Cells: State-of-the-art review. Sultan Qaboos Univ Med J. 2018;18(3):e264-e277. doi:10.18295/squmj.2018.18.03.002
Costela-Ruiz VJ, Melguizo-Rodríguez L, Bellotti C, Illescas-Montes R, Stanco D, Arciola CR, Lucarelli E. Different Sources of Mesenchymal Stem Cells for Tissue Regeneration: A Guide to Identifying the Most Favorable One in Orthopedics and Dentistry Applications. Int J Mol Sci. 2022 Jun 6;23(11):6356. doi: 10.3390/ijms23116356.
Davatchi F, Sadeghi Abdollahi B, Mohyeddin M, Nikbin B. Mesenchymal stem cell therapy for knee osteoarthritis: 5 years follow-up of three patients. Int J Rheum Dis. 2016;19(3):219-225. doi:10.1111/1756-185X.12670
Lindsay SL, Barnett SC. Therapeutic Potential of Niche-Specific Mesenchymal Stromal Cells for Spinal Cord Injury Repair. Cells. 2021;10(4):901. Published 2021 Apr 14. doi:10.3390/cells10040901
Li J, Wu Z, Zhao L, et al. The heterogeneity of mesenchymal stem cells: an important issue to be addressed in cell therapy. Stem Cell Res Ther. 2023;14(1):381. Published 2023 Dec 20. doi:10.1186/s13287-023-03587-y
Prakash N, Kim J, Jeon J, Kim S, Arai Y, Bello AB, Park H, Lee SH. Progress and emerging techniques for biomaterial-based derivation of mesenchymal stem cells (MSCs) from pluripotent stem cells (PSCs). Biomater Res. 2023 Apr 18;27(1):31. doi: 10.1186/s40824-023-00371-0.
Yen BL, Liu KJ, Sytwu HK, Yen ML. Clinical implications of differential functional capacity between tissue-specific human mesenchymal stromal/stem cells. FEBS J. 2023;290(11):2833-2844. doi:10.1111/febs.16438