Cell culture, a groundbreaking discovery made by American biologist Ross Harrison in 1907, revolutionised scientific research. Harrison has successfully cultured frog nerve cells using a hanging drop technique on a thin layer of soft agar, revealing the early utilisation of soft matrices in cell growth. Over the years, researchers devised simpler methods for growing cells on rigid glass and polystyrene tissue culture plastics, improving the efficiency of cell culture techniques. This approach has been the predominant method employed for decades.
However, it wasn’t until 1972 that scientists began noticing a remarkable phenomenon: cells exhibited distinct characteristics when grown in a soft matrix like collagen gel. This intriguing observation prompted further exploration into the influence of extracellular matrix (ECM) and substrate stiffness on cell behaviour and morphology. Consequently, significant investigations were launched to comprehend the implications of matrix stiffness in cell culture and its impact on diverse biological processes.
By understanding the role of matrix stiffness, we can unravel the intricate relationships between cells and their microenvironment, leading to advancements in fields such as tissue engineering, regenerative medicine, and disease modelling. Join us as we uncover the fascinating world of matrix stiffness in cell culture and its profound effects on cellular behaviour.
Understanding Matrix Stiffness:
Matrix stiffness refers to the rigidity or softness of the substrates, a mechanical property inherits from the ECM. The stiffness of the ECM is determined by various factors, including the composition and organization of its components, such as collagen, elastin, proteoglycans, and other structural proteins. These components contribute to the physical characteristics of the matrix and affect its mechanical properties.
Matrix stiffness is often quantified in terms of Young’s modulus, which represents the measure of stiffness or elasticity of a material. Young’s modulus reflects the relationship between stress (force applied to an area) and strain (resulting deformation) in the matrix.
Different tissues and organs in the body have varying levels of matrix stiffness. For example, bone tissue is relatively stiff and rigid, while brain tissue is much softer and more compliant. These differences in stiffness are critical for the proper functioning of cells within their respective tissues.
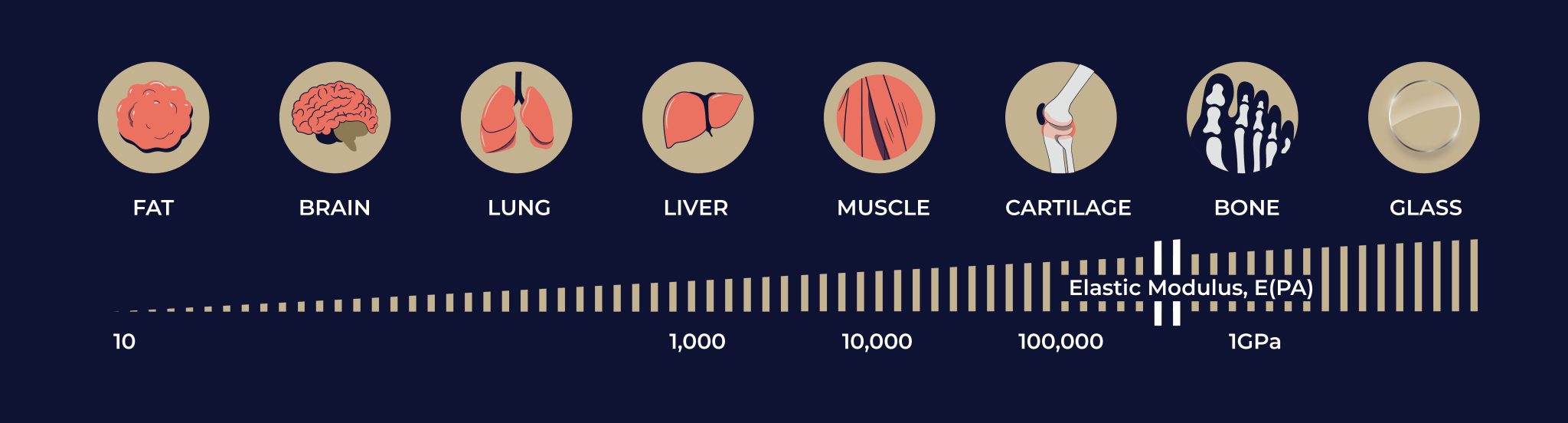
Importantly, matrix stiffness plays a vital role in regulating cell behaviour. Cells can sense and respond to the mechanical properties of their surrounding matrix through mechanosensitive signalling pathways. The stiffness of the matrix can influence processes such as cell adhesion, migration, proliferation, differentiation, and gene expression.
Impact of Extracellular Matrix Stiffness on Cellular Behaviour
Cell Adhesion and Morphology:
Cells adhere differently to matrices of varying stiffness. Generally, cells tend to spread more extensively and develop a flattened morphology on stiff substrates, while they remain rounded and exhibit limited spreading on softer substrates. This adhesion and morphological response can influence downstream cellular processes.
Cell Adhesion and Morphology:
Matrix stiffness can have a significant impact on cell proliferation and survival. One instance is a report that increasing matrix stiffness promotes proliferation and chemotherapeutic resistance, whereas a soft environment induces reversible cellular dormancy and stem cell characteristics and enhanced clonogenic capacity following chemotherapy in HCC.
Cell Migration and Invasion:
Matrix stiffness influences cell migration and invasion capabilities. Many studies have shown that ECM stiffening promotes tumour metastasis. For example, it was reported that ECM stiffening and collagen crosslinking promotes focal adhesions and drive invasion of a premalignant mammary epithelium.
Stem Cell Differentiation
Matrix stiffness plays a vital role in directing stem cell fate and differentiation. Stem cells differentiate into specific lineages depending on the stiffness of their microenvironment. In a landmark study by Engler et al., mesenchymal stem cells (MSCs) when cultured on different stiffness in the same biochemical environment differentiate into different lineages corresponding to those native tissue stiffnesses. They showed that MSCs cultured on a soft substrate (1kPa) differentiated into neural cells, while MSCs cultured on a stiff substrate (17kPa) differentiated into muscle cells, and when cultured on an even stiffer substrate (34 kPa), they differentiated into osteoblasts.
Gene Expression and Mechanotransduction
Matrix stiffness can modulate gene expression patterns. Cells sense mechanical cues from the matrix through various mechanosensor, such as integrins, focal adhesions, and cytoskeletal proteins. These signals can trigger intracellular signalling pathways, leading to changes in gene expression and ultimately influencing cell behaviour. It was reported that ECM stiffness increases expression and ESRP1-mediated alternative splicing of MENA to promote intravasation, and in another report, matrix stiffness promotes endodermal gene expression in regulating endodermal differentiation in hPSCs.
Application of Matrix Stiffness in Tissue Engineering and Disease Modeling
The understanding of matrix stiffness holds great importance in the fields of tissue engineering and disease modelling, offering numerous implications for advancing research and therapeutic applications.
In tissue engineering, the goal is to create functional and biocompatible tissues that can replace or repair damaged or diseased tissues. The mechanical properties of the ECM play a crucial role in guiding cellular behaviour and tissue development. By controlling the stiffness of synthetic matrices, researchers can design biomimetic environments that closely mimic native tissue stiffness. This is essential for successful tissue engineering applications as cells are highly sensitive to their mechanical microenvironment. Matching the stiffness of the native tissue allows cells to receive appropriate mechanical cues, leading to improved cell attachment, proliferation, and differentiation. The ability to recreate a tissue-specific stiffness gradient within engineered constructs enables the formation of functional tissues that closely resemble their native counterparts.
Moreover, various diseases, including cancer, fibrosis, and cardiovascular disorders, are characterised by alterations in the stiffness of the surrounding ECM. Tumour progression often involves changes in the stiffness of the tumour microenvironment, with increased matrix stiffness observed in many solid tumours. Abnormal matrix stiffness can impact cell behaviour, such as proliferation, migration, and invasion, and can contribute to disease progression and metastasis. In the case of fibrosis, excessive matrix deposition and tissue stiffening are key pathological features. By investigating the interplay between cells and the stiffened matrix in these disease conditions, researchers can gain valuable insights into disease mechanisms, cellular responses, and the development of potential therapeutic interventions. Understanding the influence of matrix stiffness on cell behaviour and tissue remodelling can help identify new targets for intervention and guide the development of novel treatments for these diseases.
References
Chen YF, Li YJ, Chou CH, et al. Control of matrix stiffness promotes endodermal lineage specification by regulating SMAD2/3 via lncRNA LINC00458. Sci Adv. 2020;6(6):eaay0264. Published 2020 Feb 5. doi:10.1126/sciadv.aay0264
Elsdale T, Bard J. Collagen substrata for studies on cell behavior. J Cell Biol. 1972;54(3):626-637. doi:10.1083/jcb.54.3.626
Engler AJ, Sen S, Sweeney HL, Discher DE. Matrix elasticity directs stem cell lineage specification. Cell. 2006;126(4):677-689. doi:10.1016/j.cell.2006.06.044
Levental KR, Yu H, Kass L, Lakins JN, Egeblad M, Erler JT, Fong SF, Csiszar K, Giaccia A, Weninger W, Yamauchi M, Gasser DL, Weaver VM. Matrix crosslinking forces tumor progression by enhancing integrin signaling. Cell. 2009 Nov 25;139(5):891-906. doi: 10.1016/j.cell.2009.10.027. PMID: 19931152; PMCID: PMC2788004.
Wang W, Taufalele PV, Millet M, et al. Matrix stiffness regulates tumor cell intravasation through expression and ESRP1-mediated alternative splicing of MENA [published online ahead of print, 2023 Apr 5]. Cell Rep. 2023;42(4):112338. doi:10.1016/j.celrep.2023.112338
Schrader J, Gordon-Walker TT, Aucott RL, et al. Matrix stiffness modulates proliferation, chemotherapeutic response, and dormancy in hepatocellular carcinoma cells. Hepatology. 2011;53(4):1192-1205. doi:10.1002/hep.24108