Mesenchymal stem cells (MSCs) are adult multipotent cells that have been shown to have extraordinary anticancer properties, making them a unique option for cancer treatment. Researchers are currently exploring new ways to utilize MSCs in cancer therapy, such as loading them with anti-cancer drugs, or engineering MSCs to transport medicinal compounds. However, it is important to note that there are controversial roles of MSCs in cancer therapy that should be taken into consideration.
MSCs as carriers for targeted delivery of anti-cancer drugs directly to tumours
Most anti-cancer drugs can be taken up by MSCs from their culture environment, making them a potential vehicle for drug delivery in cancer therapy. This has led to the development of simple approaches for drug priming MSCs, such as incubating them with drugs like paclitaxel, gemcitabine, or sorafenib which have been shown to be efficiently absorbed by MSCs. It should be noted, however, that the pathways for drug absorption into MSCs can vary depending on the type of drug. Some drugs are absorbed through transporters, while others rely on simple diffusion or endocytosis. This highlights the importance of understanding the specific mechanisms by which different drugs are absorbed by MSCs, as this can impact their effectiveness as drug-delivery vehicles in cancer therapy.
One of the most important therapeutic mechanisms of MSCs that have been used to produce precision medications for cancer treatment is its tumour-homing capacity. This characteristic allows for the targeted delivery of a variety of anti-cancer drugs to the site of the tumour. Upon arrival at the tumour, MSCs have the ability to release anti-cancer drugs in a time-dependent manner. The effectiveness of drug release is determined by both cell type and drug type. For example, research has shown that drug-loaded human bone marrow-derived MSCs (hBM-MSCs) begin releasing paclitaxel at a rate of 1 pg/cell after 2 hours, which increases to 1.7-2.0 pg/cell after 144 hours. On the other hand, adipose tissue-derived MSCs (AD-MSCs) were found to release most of the paclitaxel within the first 48 hours, with only small amounts released over the subsequent 144 hours.
The ability of MSCs to selectively target tumours and release drugs in a controlled manner holds significant promise for the development of more effective and precise cancer treatments.
MSCs can be genetically modified to enhance cancer therapy
MSCs have the potential to be genetically engineered to express or secrete a range of chemicals that can inhibit cancer growth and progression. There are three categories of agents that can be delivered using genetically engineered MSCs: therapeutic proteins, suicide genes, and oncolytic viruses.
Firstly, the delivery of therapeutic proteins has been proposed in many studies. Therapeutic proteins, such as growth factors, cytokines, interferons, and transcription factors, can be delivered by MSCs to inhibit tumour growth or function as inhibitors of pro-tumour factors. One such cytokine is IL-12, which can increase the activation of T cells and cytotoxic NK cells. Studies have shown that IL-12-expressing MSCs can decrease tumour growth in both renal cell carcinoma and cervical tumour mouse models. Interferons such as IFN-β, when combined with tumor-specific antibodies or standard chemotherapeutic drugs have been demonstrated to effectively limit cancer progression in mouse models. Transcription factors have also been shown to be beneficial in many studies. For example, MSCs that express the pro-apoptotic protein tumour necrosis factor-related apoptosis-induced ligand (TRAIL) have been shown to promote apoptosis in neoplastic cells in a variety of malignancies, including lung, breast, brain, cervical, and colorectal tumours.
Secondly, MSCs have been genetically modified to express suicide genes capable of converting harmless reagents into toxic anticancer drugs. This approach is based on the principle that genetically modified MSCs can convert prodrugs into their active forms in the vicinity of tumours, leading to the selective killing of cancer cells. Several enzyme-prodrug systems have been used in various types of tumours, including the herpes simplex virus thymidine kinase complexed with Ganciclovir (HSV-TK/GCV system), CD with 5-fluorocytosine (5-FC), CE with irinotecan (CPT-11), and cytochrome P450 with cyclophosphamide or ifosfamide. For example, MSCs carrying a “suicide gene,” cytosine deaminase::uracil phosphoribosyltransferase (CD::UPRT), are effective at reducing tumour growth. CD::UPRT has the ability to transform the non-toxic drug 5-fluorocytosine into the toxic anti-tumour agent 5-fluorouracil, which has been proven to be efficacious in mouse models of colon cancer and melanoma.
Lastly, oncolytic viruses (OVs) can selectively kill cancer cells by identifying and adhering to them via surface proteins, resulting in oncolysis. A variety of OVs are available, including adenoviruses, herpes viruses, measles viruses, coxsackie viruses, polioviruses, reoviruses, poxviruses, and Newcastle disease viruses. For example, oncolytic herpes simplex virus-1 has been shown to modify the tumour microenvironment (TME) by reducing the proportion of anti-inflammatory macrophages and increasing the proportion of tumour-infiltrating lymphocytes. However, the efficacy of direct OV delivery’s efficacy is typically limited since the host’s defensive mechanism can remove the exogenous viruses. As a result, MSCs are used as vehicles to transport and protect OVs.
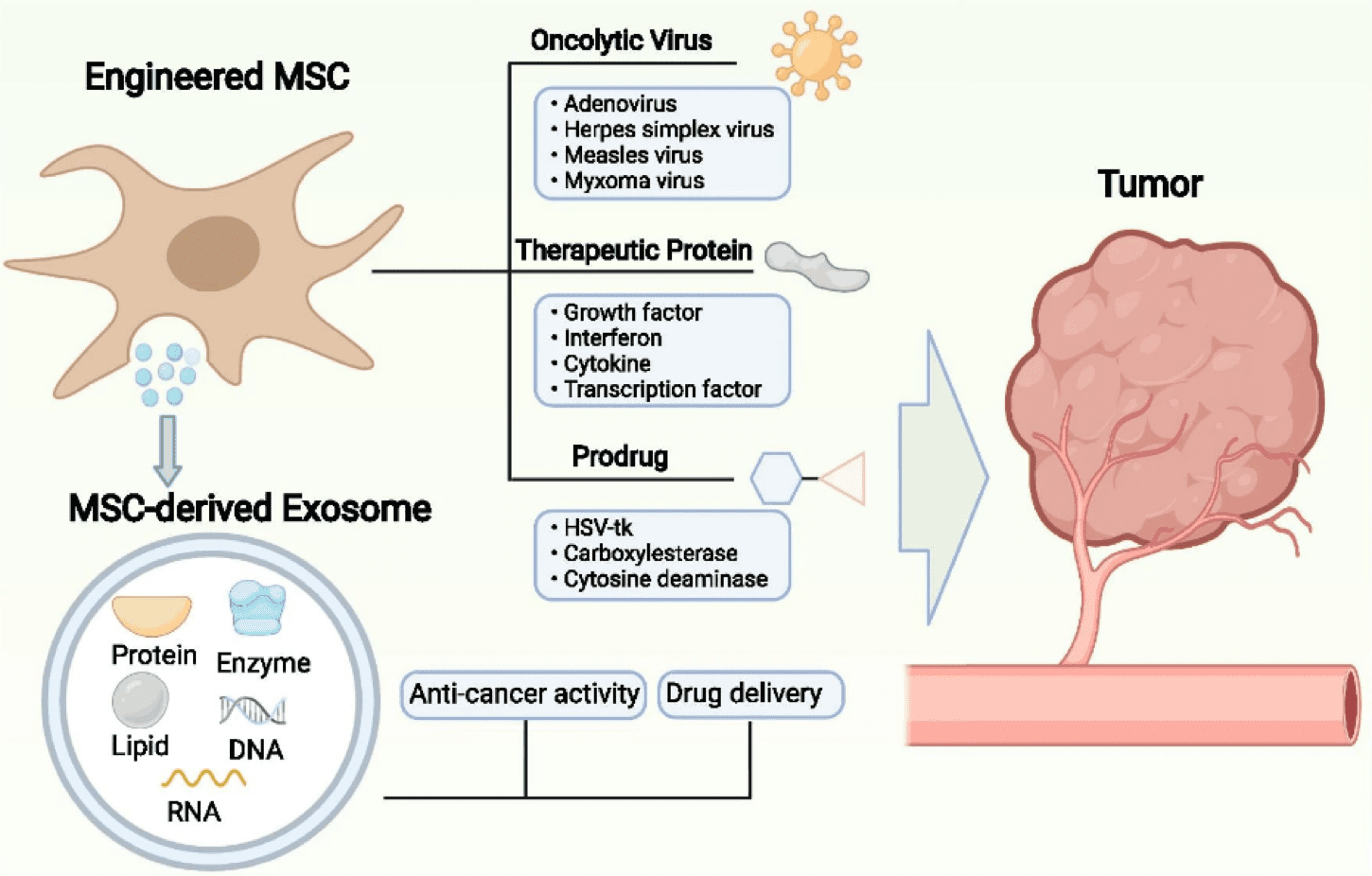
Credit: Lan T, Luo M, Wei X doi:10.1186/s13045-021-01208-w reproduced under the Creative Commons license
Controversial Roles of MSCs in cancer treatment
MSCs’ immunomodulatory properties are mediated via direct cell-to-cell interaction or paracrine release of soluble substances. However, there are several contentious MSC roles that should be mentioned, particularly regarding their impact on cancer progression.
On the one hand, MSCs may have a role in the formation and progression of cancer in various ways. For example, MSCs can impact the phenotype of tumour cells via the release of substances such as CCL5, which promotes the invasiveness of metastatic breast cancer cells. Additionally, MSCs have been linked to tumour angiogenesis by upregulating the expression levels of VEGF and IL-6 inside TMEs of breast and prostate malignancies, resulting in increased tumour vascularization. Furthermore, in response to soluble substances generated by cancer cells such as TGF-1, MSCs may develop into cancer-associated fibroblasts (CAFs). Finally, in addition to displaying pro-tumour features via soluble signalling molecules, MSC may directly promote tumour development via cell-to-cell contact or cell engulfment.
On the other hand, a large number of research has found that MSCs inhibit tumour development and progression via cytotoxicity of MSC on tumour cells. For example, previous studies reveal that MSCs cause apoptosis and reduce glioma cell proliferation via PI3K/AKT pathway. Furthermore, cell-to-cell contact causes MSC to stop the cell cycle of lymphoid cancer in the G0/G1 phase.
The ability of MSCs to suppress or increase cancer formation may be affected by differences in experimental conditions such as animal models, cell lines, dosages, and treatment durations. Therefore, it is necessary to conduct further in vivo studies to systematically investigate these roles of MSCs.
To summarize, while there are numerous promising approaches for treating cancer using drug-loaded or genetically engineered MSCs, there are also several potential concerns that must be addressed. First, the residual modified MSCs left after neoplastic cell eradication may produce unforeseen issues. Second, the therapeutic proteins delivered by MSCs may affect MSC proliferation and raise the chance of teratoma formation. Third, the efficacy of MSC tumour homing can be compromised by insufficient expression of homing molecules, which may result in off-target problems. As such, there is a significant opportunity for researchers to continue refining MSC-based techniques for cancer treatment in order to minimize these concerns and maximize their therapeutic potential.
References
Babajani A, Soltani P, Jamshidi E, Farjoo MH, Niknejad H. Recent Advances on Drug-Loaded Mesenchymal Stem Cells With Anti-neoplastic Agents for Targeted Treatment of Cancer. Front Bioeng Biotechnol. 2020;8:748.
Harrell CR, Volarevic A, Djonov VG, Jovicic N, Volarevic V. Mesenchymal Stem Cell: A Friend or Foe in Anti-Tumor Immunity. Int J Mol Sci. 2021;22(22):12429.
Hemminki O, Dos Santos JM, Hemminki A. Oncolytic viruses for cancer immunotherapy. J Hematol Oncol. 2020;13(1):84.
Lan T, Luo M, Wei X. Mesenchymal stem/stromal cells in cancer therapy. J Hematol Oncol. 2021;14(1):195.
Liang W, Chen X, Zhang S, et al. Mesenchymal stem cells as a double-edged sword in tumour growth: focusing on MSC-derived cytokines. Cell Mol Biol Lett. 2021;26(1):3.
Marofi F, Vahedi G, Biglari A, Esmaeilzadeh A, Athari SS. Mesenchymal Stromal/Stem Cells: A New Era in the Cell-Based Targeted Gene Therapy of Cancer. Front Immunol. 2017;8:1770.